Cosmos: Our Familiar Matter
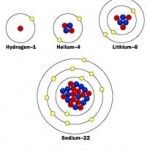
The type of matter that is made up of atoms is called baryonic matter. Some of this matter we can see, but much of it is either outside the range of visible light, or too small or too distant to see. What we can't perceive directly can often be viewed with the aid of special instruments such as telescopes and microscopes.
All baryonic atoms consist of a nucleus and surrounding layers of electrons. The nucleus consists of positively charged protons and neutrons with no charge. The electrons that spin around the nucleus are negatively charged. An atom's chemical identity is specified by the number of neutrons, protons and electrons it contains. For example, hydrogen has only one proton and one electron; helium has two protons, two neutrons and two electrons. Most elements have variations called isotopes; these structures differ usually in the loss or addition of an electron.
The two most abundant elements, hydrogen and helium, were the first to evolve. Their nuclei formed just after the Big Bang in a process called Big Bang nucleosynthesis. Some 380,000 years later, in a cooler universe, hydrogen and helium nuclei were able to bond with free electrons to become the first atoms. Those aggregated atoms became the first gas clouds in the universe and the basis for all future baryonic matter. Today, hydrogen accounts for some 75 percent of the baryonic matter in the universe, and helium for about 24 percent.
The other baryonic elements were produced by the life cycle of succeeding generations of stars in a process called stellar nucleosynthesis. Stellar fusion converts basic elements to heavier ones. The heaviest of all are produced only in the most extreme ranges of heat and pressure, the spectacular explosions of supernovas. And it is now thought a few of the heaviest, such as gold, are produced only in collisions of neutron stars.
Astrophysicists are able to calculate how much mass the universe should contain, and it is clear that baryonic matter accounts for only a miniscule portion of the total --about 4 percent. Most of the mass of the universe, some 96 percent, is thought to be a combination of dark matter and dark energy. Both dark matter and dark energy are understood only in terms of their effects rather than their source or structure.
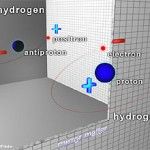
Antimatter. Every particle has an identical but mirror-image twin. For example, the electron, with negative charge, has its antimatter counterpart, the positron, which is identical except that the positron has positive charge. When a particle and its antiparticle meet, they annihilate each other, producing a burst of pure energy.
At the time of the Big Bang, when subatomic particles were created, each particle had an opposite. That was called symmetry. Obviously, in such a situation matter would have self-annihilated just as it was being created. Fortunately the symmetry was somehow broken and matter prevailed over antimatter. In principle, however, antimatter might have triumphed, in which case we would have a rather different universe. How the original symmetry was broken is unknown. Some antimatter survives in the known universe, but it is rare. It has important uses, among them the sophisticated technology of PET scans in modern medicine. July 2004 capture of antiparticle
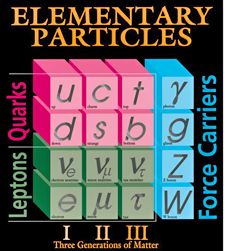
The Standard Model. As physicists penetrated the tiny universe of sub-atomic particles --the components of the neutron and proton--they discovered a bewildering array of particles they named the "particle zoo." Over time, a pattern took shape: the particles were organized into groups, and the groups came to represent a complete structure of baryonic matter, which is called the Standard Model.
The Standard Model has been very useful in explaining the elemental structure of matter, but it cannot explain why there is so much variation in the masses of the particles. This question and the question of what mass is, where it comes from, are major concerns of particle collider projects: the Fermi Lab in Illinois (shut down in 2011) and the largest machine ever built -the CERN Large Hadron Collider in Switzerland. The LHC discovery in July, 2012 of the Higgs boson will give a much better explanation of mass.